
Nitrogen Fertilizers
Anhydrous ammonia (NH3) is the most basic form of N fertilizer. Ammonia, a gas at atmospheric pressure, must be compressed into a liquid for transport, storage, and application. Consequently, it is applied from a pressurized tank and must be injected into the soil to prevent its escape into the air. When applied, ammonia reacts with soil water and changes to the ammonium form, NH4+.
Most other common N fertilizers are derivatives of ammonia transformed by additional processing, which increases their cost. Due to its lower production costs, high N content (82%) that minimizes transportation costs, and relative stability in soils, anhydrous ammonia is the most widely used source of N fertilizer for corn production in North America.
Urea is a solid fertilizer with high nitrogen content (46%) that can be easily applied to many types of crops and turf. Its ease of handling, storage and transport; convenience of application by many types of equipment; and ability to blend with other solid fertilizers has made it the most widely used source of N fertilizer in the world.
Urea is manufactured by reacting CO2 with NH 3in two equilibrium reactions:
2NH3 + CO2 → [NH4]NH2CO2 (ammonium carbamate)
[NH4]NH2CO2→ CO(NH2)2 + H 2O (urea + water)
The urea molecule has two amide (NH2) groups joined by a carbonyl (C=O) functional group.
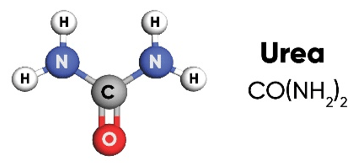
Urea-ammonium nitrate (UAN) solutions are liquid fertilizers made by dissolving urea and ammonium nitrate (NH4NO3) in water. The composition of common N solutions is shown in Table 2 and Table 3.
Table 2. Total N content and quantities of urea, ammonium nitrate, and water in 100 lbs of common UAN solutions.
UAN-28 | UAN-30 | UAN-32 | |
---|---|---|---|
Total N | 28% | 30% | 32% |
— approx. lbs in 100 lbs of solution — | |||
Urea | 30 | 32 | 35 |
NH4NO3 | 40 | 43 | 45 |
Water | 30 | 25 |
20
|
As Table 3 indicates, ½ of the total N in UAN solutions is amide N (NH2-) derived from urea; ¼ is ammonium N (NH4+) derived from ammonium nitrate, and ¼ is nitrate N (NO3-) derived from ammonium nitrate.

Figure 1. Nitrification process, showing key bacterial species and enzymes. Click here or on the image above for a larger view.
Table 3. Percent of nitrogen by type in UAN solutions.
UAN-28 | UAN-30 | UAN-32 | |
---|---|---|---|
Total N Content | 28% | 30% | 32% |
——————— % ——————— | |||
Amide (NH2-) | 14 | 15 | 16 |
Ammonium (NH4+) | 7 | 7.5 | 8 |
Nitrate (NO3-) | 7 | 7.5 | 8 |
Although there are several other forms of nitrogen fertilizers such as ammonium sulfate, calcium nitrate, and diammonium phosphate, over 80% of the N needs of corn in North America are met by anhydrous ammonia, urea, and UAN solutions.
Nitrogen Fertilizers & Soil Reactions
Anhydrous Ammonia
Anhydrous ammonia is applied by injection 6-8 inches below the soil surface to minimize the escape of gaseous into the air. NH3 is a very hygroscopic compound and, once in the soil, reacts quickly with water and changes to the ammonium (NH4+) form. As a positively charged ion, ammonium binds with negatively charged soil constituents including clay and organic matter. Nitrogen in the ammonium form is held on the soil exchange complex and is not subject to movement with water.
Soil reactions– Ammonium ions are converted to the nitrate (NO3-) form by the action of soil bacteria in a process known as nitrification (Figure 1). Nitrification is a two-step process: 1) oxidation of ammonia (NH3) into nitrite (NO2-), and 2) oxidation of nitrite into nitrate (NO3-). Both steps are carried out by chemoautotrophic bacteria in the soil that use the oxidation of chemical compounds as a source of energy. These bacteria are ubiquitous in most agricultural, pastoral, natural grassland, and forested geographies worldwide (Rajendran 2011). There are numerous species of ammonia-oxidizing bacteria; the most documented of which in agricultural systems are those belonging to the genera Nitrosomonas and Nitrosospira.Oxidation of nitrite to nitrate is carried out by bacteria in the genus Nitrobacter.
As with nearly all biological reactions, the rate of nitrification is greatly influenced by soil temperature. In soils above 75°F, (24°C) nitrification is not limited by temperature. Cold soil temperatures slow nitrification, with the process essentially ceasing at soil temperatures below 40°F (4°C).
Soil pH, water content, and oxygen availability are also major factors influencing the rate of nitrification. The optimal pH range for nitrification is between 6.5 and 8.8. Nitrification rates are reduced in more acidic soils. High pH soils are limiting for the second step of the process (oxidation of nitrite to nitrate), which can lead to a buildup of nitrite in the soil. Since both water and oxygen are required for nitrification, adequate but not excessive soil moisture is ideal. Nitrification is limited when the saturation of soil pore space with water exceeds 60%.
Only after the nitrification process has converted ammonium to negatively charged nitrate ions (that are repelled by clay and organic matter in the soil complex) can nitrogen be lost from most soils by leaching or denitrification. Plants can take up nitrogen in both the ammonium and nitrate forms. If nitrogen can be held in the ammonium form until it is taken up by plants, it is at little risk of loss. (Sandy soils with a very low cation exchange capacity (CEC) are an exception, as they lack enough exchange sites to bind much ammonium.)
Urea
Urea readily dissolves in water, including soil water; consequently, it can be incorporated into the soil by sufficient rainfall or irrigation (½ inch is typically suggested). Otherwise, it should be incorporated by tillage to reduce losses.
Soil Reactions– Urea is hydrolyzed into one carbon dioxide and two ammonia molecules (Figure 2).

Figure 2. Urea is hydrolyzed by soil bacteria producing one molecule of CO2 and two NH3 (ammonia) molecules.
Urea hydrolysis is catalyzed by urease, an enzyme that is produced by many types of bacteria and some plants and is ubiquitous in soils. The biological degradation of urea by urease that releases the N for plant use also makes it subject to volatilization (as NH3) depending on whether the reaction occurs in the soil or on the soil surface. If it occurs within the soil, the ammonia quickly reacts with soil water to form NH4+, which is then bound to the soil. If it occurs at the soil surface, the gaseous ammonia can easily be lost into the air. If plant residue is abundant on the soil surface, it increases bacterial populations, concentration of urease and volatilization losses of urea.
UAN Solutions
Urea-ammonium nitrate (UAN) solutions are mixtures of urea, ammonium nitrate, and water in various proportions. All common UAN solutions (28%, 30% and 32%) are formulated to contain 50% of actual N as amide, (from urea), 25% as ammonium (from ammonium nitrate), and 25% as nitrate (from ammonium nitrate).
Soil Reactions– The urea portion of UAN solutions reacts just as dry urea does (see previous section on urea). If applied on the surface, the amide-N in the solution may incur losses due to volatilization when urease hydrolysis releases NH3. But if UAN is incorporated by tillage or sufficient water, the NH3 quickly reacts with soil water to form NH4+. This ammonium, as well as the ammonium N derived from ammonium nitrate in the solution, adheres to soil components at the application site and is not subject to loss in the short term. Like N applied as anhydrous ammonia, this N will eventually be taken up by plants in the ammonium form, or if not, eventually converted to nitrate by soil bacteria.
The remaining 25% of nitrogen in UAN solutions is in the nitrate (NO3-) form. Because it is negatively charged, it will not adhere to clay and organic matter particles (which are also negatively charged) but rather, will exist as an anion in the soil solution. Because it moves with water, it is easily taken up by plant roots but is also subject to losses by leaching and denitrification.
Nitrogen Losses
Nitrogen loss constitutes a major challenge to agricultural efficiency and sustainability. Globally, less than half of the nitrogen applied to cropland is taken up by the crop (Zhang et al., 2015). Not only is this economically wasteful, but the loss of reactive nitrogen from agricultural soils is also associated with several adverse environmental consequences, including contamination of ground and surface water, algal blooms in lakes and rivers, hypoxic dead zones in coastal waters, and nitrous oxide emissions into the atmosphere.
Nitrous oxide from soil is the largest contributor to agricultural greenhouse gas emissions (U.S. EPA, 2021). The majority of nitrous oxide emissions from soils are produced during denitrification. Denitrification is a microbially facilitated process where nitrate (NO3−) is reduced and converted to N2 gas through a series of intermediate steps (Figure 3). When nitrate is not completely converted to N2gas, the resulting byproduct is nitrous oxide (N2O).
Figure 3. Denitrification process, showing steps and key enzymes. Click here or on the image above for a larger view.
Denitrification occurs when nitrogen in the nitrate form is present in the soil and oxygen availability is limited in the soil due to water saturation. When oxygen in the soil is limited, a variety of bacteria will use the oxygen atoms from nitrate molecules for respiration. Denitrification is triggered by rainfall events of sufficient volume to saturate at least 60% of soil pore space. The greatest nitrogen losses through denitrification generally occur in the spring when rainfall events are most frequent and crop uptake of nitrogen from the soil is relatively low.Denitrification occurs when water saturation limits the availability of oxygen to bacteria in the soil.
Nitrogen Stabilizers
Nitrification Inhibitors
Nitrification inhibitors are compounds that slow the conversion of ammonium to nitrate, prolonging the period of time that nitrogen is in the ammonium form and reducing nitrogen loss from the soil. Several compounds have proven effective for this purpose, including nitrapyrin, dicyandiamide (DCD), and ammonium thiosulfate.
Nitrapyrin, or 2-chloro-6-(trichloromethyl) pyridine, works by inhibiting and depressing the activity of Nitrosomonas bacteria; specifically, it inhibits the activity of ammonia monooxygenase (AMO), the enzyme that oxidizes NH4 into NH2OH in the first step of nitrification (Figure 4). When used in agricultural soils at labeled rates, nitrapyrin exhibits bacteriostatic activity on the Nitrosomonas population in the zone of application (Rodgers and Ashworth 1982). Inhibition of the AMO enzyme by nitrapyrin delays nitrification activity for several weeks to months following application.
Figure 4. Nitrapyrin delays nitrification by inhibiting ammonia monooxygenase in Nitrosomonas bacteria, the enzyme that catalyzes the first step of the nitrification process.
As the nitrapyrin degrades over time, AMO is no longer inhibited and Nitrosomonas populations resume the nitrification process converting available ammonia to nitrate. In warm soils, nitrapyrin can degrade in about 30-40 days. However, it is very persistent in cool soils, which contributes to its effectiveness for fall and winter applications. Measurable activity against Nitrosomonas often occurs for about six to eight weeks in warm soils conducive to crop growth, and 30 weeks or more in cool soils typical of late fall and winter in the midwestern U.S. (Trenkel, 2010).
Nitrapyrin products for delaying nitrification of ammoniacal and urea fertilizers include N-Serve® and Instinct NXTGEN®. N-Serve nitrogen stabilizer is an oil-soluble formulation of nitrapyrin for use with anhydrous ammonia. Instinct NXTGEN nitrogen stabilizer is a water-based microencapsulated formulation of nitrapyrin that may be used with urea, UAN solutions, ammonium sulfate, liquid manure, aqua ammonia, liquid fertilizers containing N, and ammonium-containing dry fertilizers (MAP or DAP).
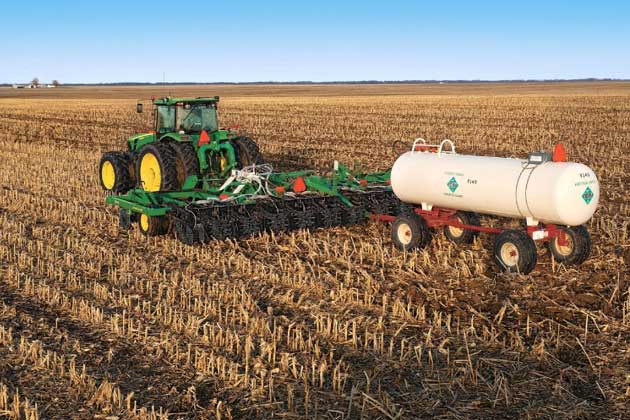
DCD (dicyandiamide) -Following extensive use in Western Europe and Japan, DCD became more commonly used in the US in the late 1990s. Products containing only DCD are generally used with nitrogen solutions and liquid manure. The rate of DCD used is relative to the amount of fertilizer N applied, rather than the area of application. This may limit its efficacy at low fertilizer application rates (e.g., split applications, side-dress applications, or crops that require low nitrogen rates).
DCD inhibits nitrification in the same way as nitrapyrin, by inhibiting the activity of ammonia monooxygenase in Nitrosomonas bacteria. However, DCD is a significantly less potent inhibitor, requiring higher field use rates to be effective and inhibiting nitrification for a shorter period of time. Depending on the amount of mineral N applied and the moisture and temperature of the soil, DCD may stabilize ammonium-N for about 4-10 weeks.
Value of Nitrification Inhibitors
Nitrification inhibitors have proven very effective in increasing soil nitrogen retention and reducing losses through leaching and denitrification. A 2004 meta-analysis of hundreds of comparisons across a diversity of environments found that the use of nitrification inhibitors increased soil nitrogen retention by an average of 28% and reduced leaching by 16% (Wolt, 2004). Nitrous oxide emissions were reduced by over 50% on average in this study, indicating that nitrification inhibitors can be a valuable tactic for reducing agricultural greenhouse gas emissions.
Corteva Agriscience field trials conducted over several years found that the use of nitrification inhibitors increased corn yield by an average of around 6 bu/acre. The highest value of nitrification inhibitors should be realized in scenarios with a high risk of nitrate losses from leaching or denitrification, including the following conditions (Ruark, 2012):
- Tile-drained soils when leaching potential is high
- Wet or poorly drained soils
- Fields with nitrogen applied in the fall or spring prior to planting
Urease inhibitors are compounds that reduce volatilization losses of urea applied to the soil surface by slowing down urea hydrolysis. For the nitrogen in urea to be available to plants, it must undergo hydrolysis, a chemical reaction that transforms the amide groups of the urea molecule into ammonia (NH3). The urease enzyme, ubiquitous in soils, catalyzes this hydrolysis reaction. If this process occurs at the soil surface, ammonia can be lost to the air. However, if this reaction is delayed until surface-applied urea is incorporated into the soil by tillage, rainfall, or irrigation, the risk of ammonia loss is greatly reduced.

Urea granules on the soil surface next to corn plants at the V4 growth stage. Urea that is not incorporated can be lost to volatilization without the use of a urease inhibitor.
Urease activity increases as temperature increases. Hydrolysis is normally completed within ten days at a temperature of 40°F (4°C) and within two days at a temperature of 85°F (29°C). Hydrolysis is also highly correlated with the organic matter, total N and cation exchange capacity (CEC) of the soil; increasing as any of these factors increase.
Certain compounds are known to inhibit the hydrolytic action of the urease enzyme, delaying urea hydrolysis. The most widely used urease inhibitor in agriculture is N-butyl-thiophosphoric triamide, (NBPT). NBPT is a structural analog of urea and, as such, inhibits urease by blocking the active site of the enzyme. NBPT is the active ingredient in PinnitMax® TG nitrogen stabilizer.
PinnitMax TG is an additive for use with urea and urea-ammonium nitrate solutions. Research shows that N loss from surface-applied urea can be significant. The amount of loss depends on weather conditions; loss is greatest with warm, windy weather and a moist soil surface. NBPT protects urea and UAN applications from volatilization for up to 14 days, helping ensure nitrogen gets to the plant root zone. Eventually, NBPT degrades in the soil, allowing urea hydrolysis to resume. This is necessary so that plants can take up and use the nitrogen from urea. However, once in the NH4+ form, this nitrogen is subject to nitrification to NO3- a form that may be lost from the soil.
Performance of Nitrogen Stabilizers
Nitrogen stabilizers/additives have been widely tested over many years and have proven effective at increasing soil nitrogen retention. However, corn yield increases can vary from 0-20%. This is not surprising; when conditions favor nitrogen losses for a period, and a stabilizer is applied and effective during that period, a large benefit is predictable. On the other hand, under conditions not conducive to nitrogen losses, little advantage would be expected.
Because the risk of nitrogen loss is always present, growers should take appropriate precautions to reduce the loss of this important crop nutrient. This can be accomplished by picking an appropriate nitrogen source and applying it as closely as possible to the time of crop uptake or by using a nitrogen stabilizer when application timing is farther removed from the period of crop need. Nitrogen management decisions should take into account all factors that influence the risk of loss for a particular field, including local climatic conditions, topography, soil type, residue level, form of nitrogen fertilizer applied, and timing of application relative to crop growth. Nitrogen stabilizers can provide insurance against the risk of nitrogen losses in many susceptible fields.
Post a comment
Report Abusive Comment